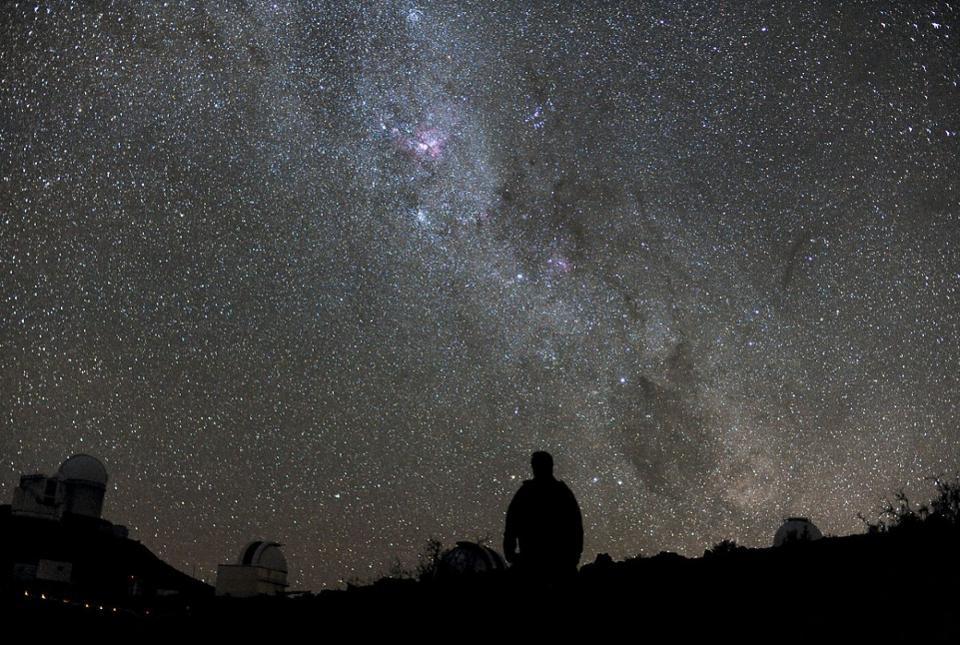
When you look out at a star whose light arrives after traveling toward you for 100 years, you’re seeing a star that’s 100 light years away, due to the fact that the speed of light is finite. But when you look out at a galaxy whose light arrives after traveling toward you for a journey of 100 million years, you’re not looking at a galaxy that’s 100 million light years distant. Rather, you’re seeing a galaxy that’s significantly farther away than that!
The reason for this is that on the largest scales — objects that aren’t gravitationally bound together into galaxies, groups or clusters — the Universe is expanding. The longer it takes a photon to travel from a distant galaxy to your eyes, the greater the role of the Universe’s expansion, implying that the most distant galaxies are even farther away than the amount of time the light from them has been traveling.
This shows up as a cosmic redshift. Since light is emitted with a particular energy, and hence a particular wavelength, we fully expect that it will arrive at its destination with a particular wavelength as well. If the fabric of the Universe were neither expanding nor contractin
g, but rather were constant, that wavelength would be the same. But if the Universe is expanding, the fabric of that space is stretching as shown in the video above, and hence the wavelength of that light becomes longer. The great redshifts we’ve observed for the most distant galaxies have absolutely verified this picture.
But we can do much more than determine that the Universe has expanded and continues to expand. We can use all the information we gather to conclude how the Universe has expanded over its history, which in turn tells us what the Universe is composed of.
Once the light leaves a distant, cosmic source, the expanding Universe stretches the wavelength of that light. This leads to a redshift, where the more distant objects will have their light redshift for longer amounts of time, when different components of the Universe (like dark energy, matter, or radiation/neutrinos) were more important.
By measuring sources at a whole slew of distances, discovering their redshift and then either measuring their intrinsic vs. apparent size or their intrinsic vs. apparent brightness, we can reconstruct the entire expansion history of the Universe.
In addition, since the way the Universe expands is determined by the various types of matter and energy present within it, we can learn what our Universe is made out of:
- 68% dark energy, equivalent to a cosmological constant,
- 27% dark matter,
- 4.9% normal (protons, neutrons and electrons) matter,
- 0.1% neutrinos and antineutrinos,
- about 0.008% photons, and
- absolutely nothing else, including no curvature, no cosmic strings, no domain walls, no cosmic textures, etc.
- Once we know the components of the Universe to this degree of precision, we can simply apply this to the laws of gravity (given by Einstein’s General Relativity), and determine the future fate of our Universe. What we discovered, when we first applied this to the discovery of a dark energy-dominated Universe, was shocking.
First off, it meant that all the galaxies that weren’t already gravitationally bound to us would eventually disappear from view. They would speed away from us at an ever-increasing rate as the Universe continued to expand and expand and expand, unchecked by gravitation or any other force. As time went on, a galaxy would get more distant, meaning that there was an increasing amount of space between that galaxy and ourselves. The galaxy appears to move away at greater and greater speeds, due to the expansion of space.
- But this leads to an inevitable conclusion that is even more disturbing. It means that, at a particular, key distance from us, due to the expansion of the fabric of space itself, a photon either leaving our galaxy toward a distant one or approaching ours from a distant galaxy will never reach us. The expansion rate of the Universe is so great that distant galaxies become unreachable to our own, even if we were to move at the speed of light!